Exciting developments in the field of oligonucleotide drugs
Jonathan Hall shares his perspective on the history of oligonucleotide drugs, collaborations with industry, Knowledge & Technology Transfer and describes his career path.
Oligonucleotide drugs have a relatively young history compared to small molecule drugs and antibodies. Could you give us a short overview of the history and the milestones of oligonucleotide drugs from your perspective?
The history of oligonucleotide drugs begins in the late seventies, early eighties. It was recognized, that oligonucleotides bind to complementary RNAs with a fantastic selectivity and very high affinities. That made it possible to consider RNA as a potential new class of therapeutics. To drive this forward, a lot of work was carried out in a small number of groups in academia and biotech and at the same time real advances in the oligonucleotide synthesis on the solid phase were starting to take off. Although it was clear that these types of reagents could work very well in vitro (cell culture), the step towards in vivo use of oligonucleotides was going to involve really big challenges; On the side of the chemistry, on the pharmacology, but also on the side of disease biology. These challenges were met stepwise, working in parallel, and it was helped along by the launch of a handful of oligonucleotide therapeutic companies: Companies like Ionis Pharmaceuticals [formerly Isis Pharmaceuticals; for the rest of the interview the name Ionis is used], Gilead Sciences or Hybridon, to name a few. The discovery that phosphorothioated RNAs circumvented some of the pharmacokinetic problems was certainly a milestone of oligonucleotide drug discovery and development. Another was the discovery of the RNase H mechanism, whereby it was recognized that most oligonucleotides working in cells and also in vivo actually induced enzyme-mediated degradation of the mRNA. The approval of the first drug in 1998, Fomivirsen [trade name Vitravene®], which was injected directly into the eyes of AIDS patients suffering from cytomegalovirus [CMV] infections, was an important milestone. Although the drug had non-specific effects and it was a commercial failure, its approval forced the regulatory authorities to consider oligonucleotides as a new class of drugs. The molecule was withdrawn as other conventional antiviral drugs came on the market, which were more effective and orally available. The field then had to wait a further 15 years before the approval of the first good antisense drug, called Mipomersen [trade name Kynamro®]. In those intervening years the field made a lot of progress, particularly in the field of medicinal chemistry. Large pharma companies have played a significant role in the development of this field as they supported some of the early antisense companies with substantial research and development collaborations. However, by the time the first drug was approved, most of large pharma had actually stepped backed, mainly because of the slow rate of progress. It has always been tempting to compare the rate of development in oligonucleotide therapeutics to that of the antibody drugs, and oligonucleotides are taking much longer to be accepted as a viable therapeutic. However, there is no real reason why such a comparison is valid. Other important milestones were the discovery of a new class of RNA targets in the form of microRNAs (miRNAs) and at the same time, the introduction of small interfering RNAs (siRNAs). As the most recent milestone, I would consider the results from clinical trials of the Ionis drug Nusinersen to treat children affected by Spinal Muscular Atrophy. The CEO of Ionis Stanley T. Crooke showed at the Oligonucleotide Therapeutics Society meeting this year a short movie of a child that was treated with the SMN2 splice-correcting oligonucleotide Nusinersen, a molecule developed with Adrian Krainer (Scientific Advisory Board Member NCCR RNA & Disease) at CSHL. The beginning of the movie showed a baby who could basically not move his head, arms or legs, but as the baby grew into a small child we saw the progress in terms of movement capabilities after drug treatment. That was powerful! That is splicing correction with oligonucleotides. Precision medicine!
Can you give us some more insights into the different chemistries of oligonucleotides?
In the early days of oligonucleotide therapeutics much of the research was devoted to dealing with the major pharmacokinetics challenges. The problem with natural oligonucleotides is that they are degraded in vivo by nucleases extremely quickly. The challenge for medicinal chemists was therefore to find a means to protect them against nucleases, while maintaining their Watson Crick recognition properties, their ability to activate RNase H, their suitability for solid phase synthesis and keeping the structure sufficiently simple to permit large scale manufacture. Besides the phosphorothioate modification, very few chemical modifications satisfy all these criteria. Nevertheless, a handful from literally hundreds of modifications tested made it through to clinical studies. Around the mid-nineties the methoxyethyl modification was developed by the Novartis (former Ciba-Geigy) group. It was subsequently licensed by Ionis pharmaceuticals and became the mainstay of all their clinical programs. Around 2000 there was the invention of the locked nucleic acid (LNA) simultaneously by Danish and Japanese groups. This particular modification also made it as far as clinical trials. A chemical modification, which remained rather secret for a long time with respect to its structure and properties, was the morpholino modification, appearing in the mid-nineties. It has rather an innovative structure, and does not activate RNAse H but still found much use as tools to be used in model organisms. It has proved valuable in a class of oligonucleotides that work by a special mechanism, so called splice switching oligonucleotides (SSOs). Exciting developments continue to be seen: one is the introduction of chiral phosphorothioate oligonucleotides, as all oligonucleotides in clinical development are currently mixtures of diastereoisomers. The second is the so-called GalNAc conjugates, relatively large oligosaccharide ligands introduced into siRNAs, tremendously increasing delivery to the liver. The delivery of oligonucleotides is still seen as a main hurdle for this technology and it is true that oligonucleotides to date can only be used in a relatively small number of tissues, particularly liver, kidney and the central nervous system. Thus, today, a lot of academic research is devoted to the use of so called sophisticated targeting molecules to broaden the scope of the technology.
How does the chemistry of oligonucleotides affect their delivery and stability?
Oligonucleotide drugs will probably never be orally bioavailable with acceptable efficiency. They can be delivered typically intravenously or with subcutaneous injections. When phosphorothioate drugs are delivered systemically, the phosphorothioates in the molecule cause them to associate with proteins in the blood. This turned out to be a very useful property because it actually kept them in the body such that they distribute rather broadly to almost all tissues and organs. Without this protein binding they would be excreted rather quickly. The second and third generation modification chemistries make the single stranded oligonucleotide drugs rather stable against metabolic degradation compared to the first generation. The methoxyethyl modification in single stranded oligonucleotides is basically a rock. I am not aware of any reports in the literature where accumulation of these drugs in vivo has caused any problems. They are of course metabolically degraded and secreted as truncated versions of the original sequence. There is some concern that some of the modifications, particularly those in siRNAs, may lead to some long-term toxicity, as modified nucleotides are incorporated into natural polynucleotides in the body. But again, to my knowledge there is no evidence that this has actually been observed.
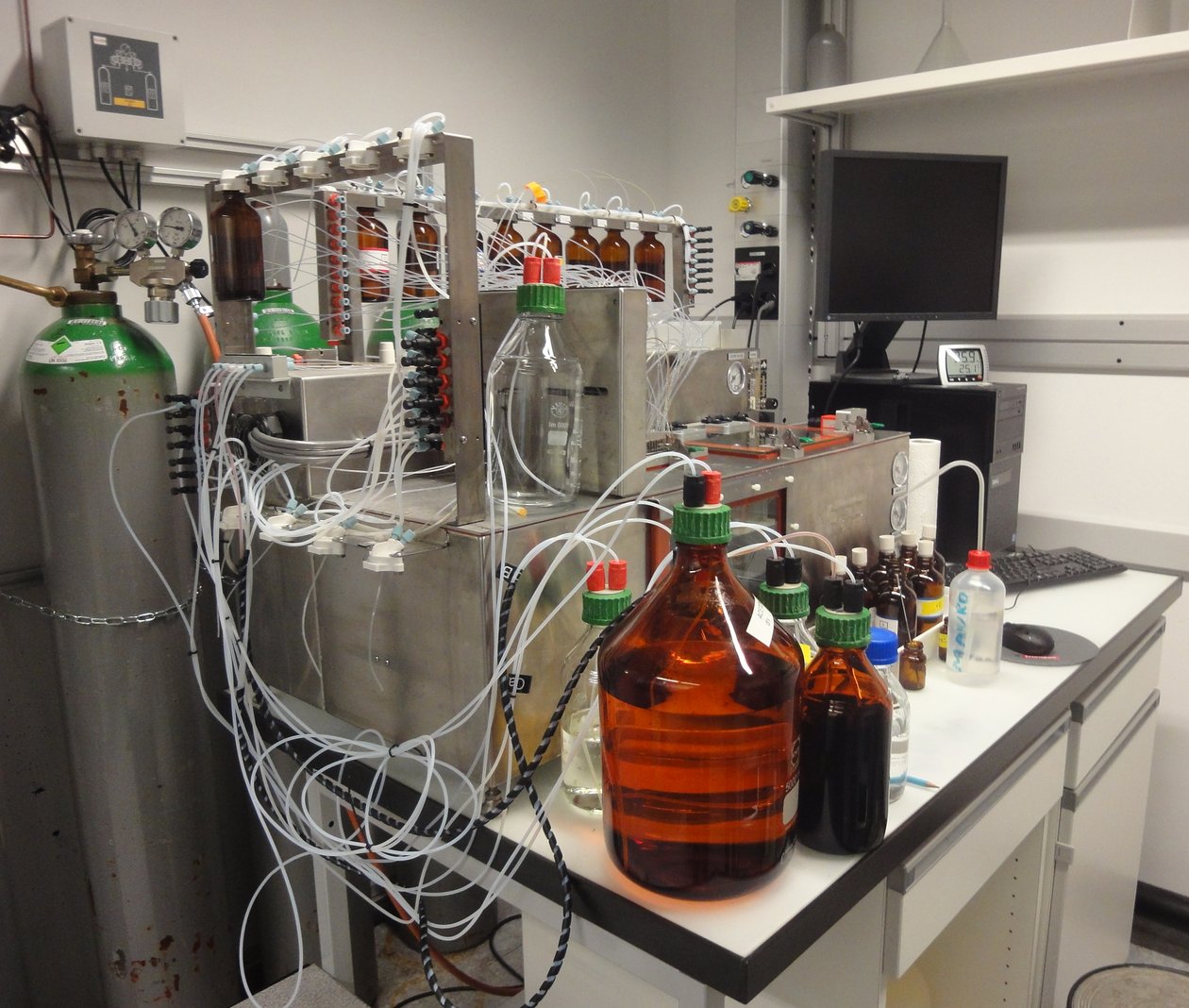
When did you start working on oligonucleotide chemistry?
I first started to work on nucleic acids in 1992 when I started at the Central Research Laboratories at Ciba-Geigy. I had never worked with nucleic acids before, but this type of chemistry, especially in terms of the molecular recognition of oligonucleotides, was extremely attractive for a chemist. At that time at Ciba-Geigy, which became Novartis in 1995, all of the scientists working in the area were chemists. In 1996 the management decided to move resources more towards the biology, particularly the disease biology in house. The fundamental make-up of the group then changed and most of the chemists were replaced by people working more on the biological applications side. Then we had a long phase through the second half of the nineties trying to find the best use of this particular technology among the various therapeutic areas in the company, e.g. neuroscience, transplantation, oncology and respiratory diseases. Around 2000, the sequence of the human genome was starting to become available and our focus switched from therapeutic applications of oligonucleotides to their use in large libraries as tools to help functionalize newly discovered genes emerging from the sequencing programs. We only really went back to the therapeutics with data that was emerging from the Novartis group in London working on neuropathic pain and when it became clear that oligonucleotides were showing exciting data for the treatment of neuropathic pain through spinal cord delivery.
How did you experience the uncertainty not knowing what the company decides to do next?
All large companies go through reorganizations from time to time and then scientists in the research organization ask themselves how the reorganization will affect them. It is particularly concerning when you are working in an area progressing relatively slowly and when there is still quite a lot of skepticism from the more conservative members of management about the potential for success of this class of drugs. Ciba was a company that was very supportive of the technology compared to other companies. Many large companies had activities in this area initially, but most of them do not have dedicated groups doing the fundamental research any more. New technologies have a natural lifetime and usually often follow such paths. In the beginning, a company will dedicate a lot of research to advance a technology in order to find out how it works best for them, and after it becomes routine then basically disband the dedicated group and use it when needed. In the case of oligonucleotides, these activities were basically shut down at large companies before the technology was validated on the market. With new approvals, which will come in the next 2-3 years, I am confident that once again interest will be triggered at the larger companies
Why does it seem that in the end small companies were more successful than the large ones in the oligonucleotide therapeutics field?
Most of the small companies that started out have gone. They have gone for a number of reasons: because their programs failed or they lost out in battles over intellectual property. The companies working on single stranded oligonucleotide drugs that have remained are basically Ionis and Santaris, which was recently bought by Roche. What distinguishes these companies from other companies has been actually the quality of their science more than anything else. They have made substantial progress on the important challenges in the field. This is also true for small interfering RNAs; the largest company working in this area being Alnylam Pharmaceuticals. I personally believe that we all underestimated the challenges associated with bringing this new pharmacology to the point where it is validated and is used on suitable drug targets for the right diseases. It is correct to say that oligonucleotides can basically target almost any RNA in the body, but only very few RNAs actually represent truly useful targets for disease indications. Being able to select those has not been so easy and thinking has evolved. In the early stages of the field, these were viral RNAs and targets involved in cancer. Now, the focus is on targets that are expressed in the liver, particularly those that have some kind of genetic validation associated with them. For commercial reasons, targets need to be free of competition from small molecules and antibodies.

Were there similar fierce patent disputes in the oligonucleotide field as there are currently taking place around the CRISPR/Cas9 technology?
Yes, in particular in the early days of the technology a large number of key patents were filed and issued: Key patents around the use of phosphorothioates, phosphoramidite reagents, the dimethoxytrityl group and oligonucleotides, which work by RNAse H mechanisms. All these patent applications were filed and were fiercely defended by the companies that held them. This was also the case with small interfering RNAs. The original patents were argued over for many years. And in my view this has, more than anything else, held up developments in the field. Certainly larger companies were reluctant to enter an area where difficult intellectual property issues existed. Constraining the number of companies that can actually work in a particular area generally slows down the progress in the field. Paradoxically, this affects even those who hold the key patents.
What made you become a scientist and were there any scientists that inspired you?
It was probably my high school teacher Mr. Hawkins, who had a very aggressive character, and he said: “Hall you will do chemistry” and so I did. I was always more interested in science than in languages or humanities. My PhD mentor (Edward Smith) was also somebody who inspired me. He maintained a very small group at Imperial College and put a lot of attention on the PhD students. He was a fantastic person to guide me through the PhD. Somebody who also had a positive effect was Professor Jean Marie Lehn at the University of Strasbourg who was my first postdoc supervisor. He is a tremendous scientist, Nobel laureate, and is able to motivate scientists in numerous ways. He was so interested in the projects and so useful when things were going badly. I think more than anything else he kept me in chemistry and in science because of the way he was, and still is.
What made you move from academic to industrial research?
At the time it was quite difficult to actually get an academic position. In those days you applied for all positions that were advertised, and academic positions were rare. I was successful in getting job offers from three large pharma companies. The science in Basel with the nucleic acids and the new head of this department, who was a truly motivating person to interact with, made that position really appealing. It was not a difficult decision to leave academia and move into industry. It was much simpler than that. I was confronted with a project and an area of research that was just extremely attractive. It also helped at the time that Ciba Central Research Laboratories was quite a well-known organization for doing high-risk, forward-looking research. It was a large department with its own budget which was protected from the more conservative company management. We were actually judged on how we published our work, we were encouraged to go to meetings and we rarely faced hold ups because of patenting issues or typical large company traits.
What triggered your move back to academic research?
It was not one single event. My current position was open at the ETH and after investigating, it became quickly clear that to start again with a new research group at this institute was truly a fantastic and wonderful opportunity. It also came at a time when I was seeing less and less of the projects being done in the Novartis labs.
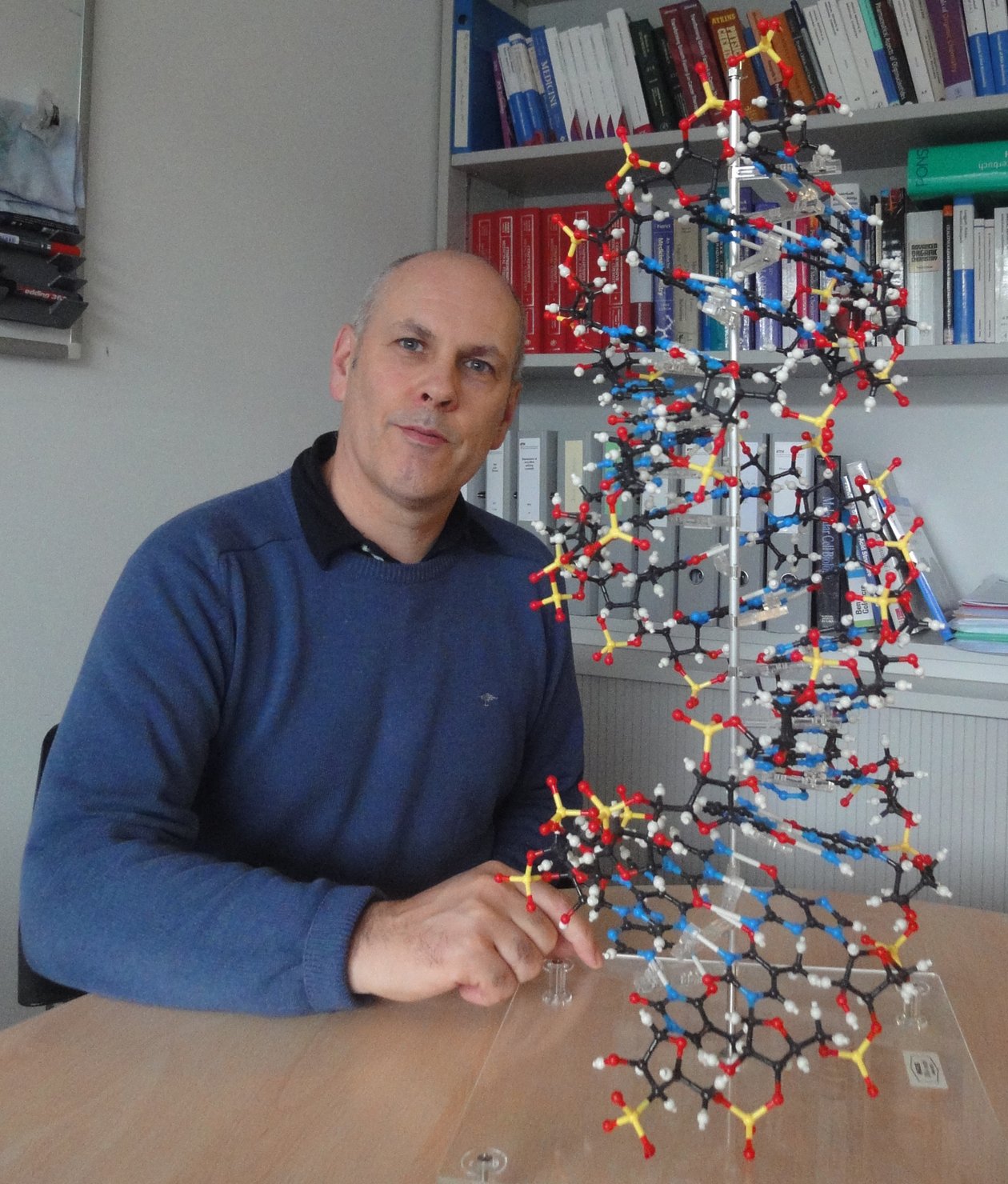
What are differences between research in academia and big pharma companies?
I spoke with several professors before moving from Novartis to here. One told me that what I would really enjoy and profit from the ETH environment because I would have the opportunity to take the time to set up the research to the highest scientific standards, setting my own timelines and priorities. That meant that I could truly focus on doing careful science in a way that one can simply not do in large pharma. The pressures of the short term deadlines preclude a lot of the types of experiments that we perform here, trying to fully understand our molecules before progressing to the next step. This is a fundamental difference. The other really large difference comes down to the people you actually interact with. In pharma we hired scientists that had already proven track records. In the academic environment we are actually training these people.
How did you interact with academia during your time in the pharmaceutical industry?
We had a lot of interactions and our own budget for collaborations, which we could use almost as we wanted. One did not have to endlessly justify collaborations to research management, nor how we set them up. These days it seems to me that it is much more difficult for the scientists in large pharma to interact with academia. The decisions to engage in even relatively small-scale collaborations seem to require a lot of discussions and signatures on various levels in the hierarchy. This tends to slow down and even prevent potential good collaborations from starting.
What are the chances and pitfalls regarding collaborations with big pharma?
I believe that your best chance to have a meaningful collaboration with industry these days is if you have a unique technology or drug target that they are uniquely interested in. In this way companies are much more direct and selective these days. I do not know about the pitfalls, but it seems to me that the legal aspects of collaborations also tend to make them much more difficult to set up. It takes forever to sort out legal agreements and often by the time that an agreement is actually signed, the motivation to actually do the project may have diminished. It seems to me also, that large companies are a lot less willing to fund academic research in a general sense, which is a shame because they lose the chance to make contacts with their future employees.
Can you comment on the importance of Knowledge and Technology Transfer (KTT) for the NCCR RNA & Disease?
The KTT aspects of the NCCR are really important. We do have a large responsibility to show Swiss society and taxpayers that we are spending their money well. Furthermore, KTT offers an opportunity to help our younger generation of researchers because one of our objectives is to stimulate the growth of companies. KTT in biomedical research is quite difficult because our progress is naturally slow compared to perhaps an engineering discipline, where one can have an idea and potentially form a company quite quickly.
What advice would you give NCCR RNA & Disease researchers regarding KTT?
We should sensitize everybody that receives funding from our NCCR to the importance of showing the value of our research to the outside world. My advice to colleagues would be to try to think about your projects in this context and search high and low for possibilities to actually bring new applications and discoveries of practical use out of your research.
Further Reading:
“RNA therapeutics: beyond RNA interference and antisense oligonucleotides”
Ryszard Kole, Adrian R. Krainer and Sidney Altman (2012)
Nature Reviews Drug Discovery, 11(2):125-40
Linkt to Pubmed (PMC Free Full Text Available)
Biography Prof. Jonathan hall
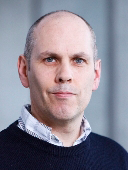
Jonathan Hall is full professor for Pharmaceutical Chemistry at the Institute of Pharmaceutical Sciences at ETH Zürich since 2007. He serves as the delegate for Knowledge and Technology transfer (KTT) of the NCCR RNA & Disease, in which he also is a principal investigator investigating the drugability of RNA. The group of Jonathan Hall runs the RNA synthesis platform of the NCCR RNA & Disease.
Jonathan Hall obtained his bachelor and PhD (supervisor: Edward Smith) degrees from the Imperial College in London. He continued his research career with postdoctoral stays in the laboratories of Jean-Marie Lehn and later Yoshito Kishi. Before joining ETH Zurich Jonathan Hall worked as a researcher and group head for fifteen years first at the Ciba-Geigy Central Research Laboratories and subsequently at the Novartis Institute for Biomedical Research. There he began to work on the chemistry of nucleic acid synthesis and their applications as therapeutics and screening tools.
Interview: Larissa Grolimund and Dominik Theler